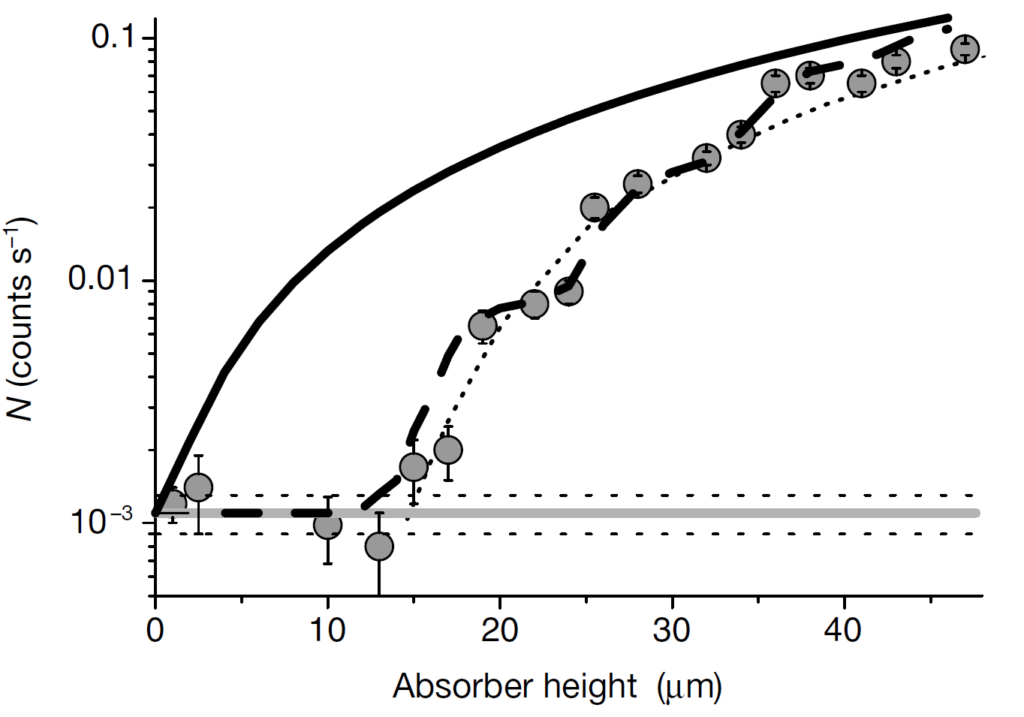
In this project, we will use Gravitational Quantum States (GQS) of light neutral ultracold particles, hydrogen H and neutrons n. GQS are formed with gravity on top and a specularly reflecting mirror with a sharply changing surface potential at the bottom. Particles move classically in the horizontal direction if the mixing of velocity components is small. As the masses of H and n are nearly equal, their energies and wave functions in GQS are also nearly the same and characterized by ultralow energies (ε0~0.6peV) and large sizes (l0~5.9μm).
GQS were discovered with n in 2002 [1-4] by a team led by V.Nesvizhevsky (see figure above from ref. 1, for references see the menu “Publications”), and since then they have been actively used by several groups (Tokyo, qBounce, GRANIT) [6-9] to constrain theories with extra dimensions, new bosons, dark matter, etc. While repulsive n-nuclei potentials [10] of materials produce reflection of ultracold n from surfaces, attractive van der Waals/Casimir-Polder (vdW/C-P) potentials also reflect ultracold atoms from surfaces due to quantum reflection [11-20]. These reflection processes have been studied in papers as early as 1936; since then they have given rise to fields of research with innumerable applications. The vdW/C-P surface potentials are also expected to reflect antiatoms [21-23] and form their GQS [25-30]. Nobody has ever observed GQS of atoms or anti-atoms. However, major motivations prompt efforts to observe and measure them with a maximum accuracy:
1. New fundamental interactions (due to new bosons or other phenomena beyond Standard Model (SM), manifestations of extra dimensions or dark matter [35-53,94]) would equally modify energies of GQS of H and n. Extreme smallness of both GQS energies (~1peV) and contributions of other fundamental interactions provide the high sensitivity of GQS to the presence of new interactions between particle and mirror. The sensitivity depends on GQS lifetimes τ and particle phase-space densities ρ. Evidently, studies with H promise a dramatic increase of ρ. Due to trapping, H will also increase τ compared to those achieved with n. Thus, studies with H promise to surpass the current sensitivity with n. Below we will address a strategy to improve n experiments as well. The same arguments hold true for centrifugal quantum states (CQS) [54], which are analogous to GQS with the centrifugal acceleration replacing gravity and a curved mirror replacing a flat one.
2. Ongoing experiments with antihydrogen atoms at CERN (AEGIS, ALPHA, GBAR) [31-33] aim to measure the gravitational acceleration of antimatter. The most precise method proposed so far relies on GQS of in future experiments [27,34,96]; a projected accuracy is ~10-4-10-6 depending on densities achieved and a chosen measuring mode. A straightforward way to prove the existence of GQS consists in performing measurements with H to explore systematics and optimizing the techniques prior to starting resources-limited studies at CERN. Thus, GRASIAN is quite timely. Moreover, the planned periods of extended shutdowns of CERN and ILL will allow the PIs to focus on this project when needed.
3. Using the methods developed with GQS, we may accept large horizontal velocities, which are not relevant for the spectroscopic techniques we will use, but provide tremendous gain in statistics.
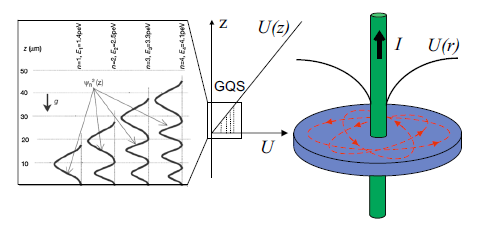
Observation time is the key parameter for improving the precision of measurements of GQS of particles levitating above a reflecting surface. As one option to increase it, we propose a new method [111] of long confinement in such states of atoms, anti-atoms, neutrons and other particles possessing a magnetic moment. The earth gravitational field and a reflecting mirror confine particles in the vertical direction.
The magnetic field originating from electric current passing through a vertical wire confines particles in the radial direction. Under appropriate conditions, motions along these two directions are decoupled to a high degree. We estimate characteristic parameters of the problem, and list possible systematic effects that limit storage times due to the coupling of the two motions.